Mission
Our mission is to build a world-class vertically-integrated global network of out-patient cancer treatment and diagnostic centres employing a methodology
that at its core utilises precision radiotherapy
to replace surgery in conjunction with
the highest standards of care in a cost-effective manner to democratise the treatment of cancer
Vision
COIL recognizes that rapid changes are currently taking place in the treatment of cancer patients posing new operational and managerial challenges to health care providers. One particularly complex area involves the implementation of advanced ablative radiation techniques, as the sole curative approach replacing surgery, or its integration with of other established and novel treatment modalities. COIL provides a strategic initiative to introduce innovative, more effective and personalized treatment modalities in the treatment of cancer.
The planned COIL facilities will consistently deploy established cutting-edge modalities largely centered, albeit not exclusively, on stereotactic body radiation therapy (SBRT) going on to include single-dose image-guided radiotherapy (SDRT). This innovative mode of operation differs from existing conventional radiotherapy centers, and is based on advanced technologies which enable sparing of non-target tissues, thereby exploiting the mechanism of local cancer cure by ultra high-dose radiation exposures.
COIL will specifically focus and provide leadership in introducing Hypofractionated Radiotherapy, IG-RT and SDRT as state-of-the-art radiation treatments for the control and cure of human cancer globally through excellence in patient care, outreach programs, clinical research, continued medical training programs. In the fulfillment of its mission, COIL will:
• Employ state-of-the-art radiation technology, while providing the best possible quality of life for its patients.
• Advance its capability in cancer patient care through a vigorous pursuit of research and rapid application of new knowledge acquired and validated through pre –clinical and clinical research carried out by AEI.
• Assure a safe environment and treatment delivery practices that are designed to minimize risk to patients and staff.
• Participate in the development and implementation of public policies related to cancer treatment through research, clinical care, patient education and public programs
• Provide effective management and administer sound policies to ensure quality, consistency and productivity of its programs in patient care, research and education and to secure their relevance to the control and cure of cancer
• Provide timely and respectful support to patients and families
Objectives
To achieve its mission goals, COIL will pursue the following objectives over its first five years of operations:
• Complete by late 2017 the acquisition of a European-based facility which will allow it
to offer sector-leading cancer-care facilities, devoted to the practice of SBRT as well
as immunotherapy;
• Assemble an international core team with practice-leading expertise in radiation
medicine and medical physics to import and supervise the implementation of the
demanding techniques of high-precision SBRT;
• Provide full training of qualified staff members in the use of SBRT in conjunction with
other established advanced imaging modalities and cancer treatment approaches.
This will occur through an agreement with AEI;
• Establish a compendium of SOPs (Standard Operating Procedures) for clinical
protocols and quality assurance procedures to be implemented globally in all COIL facilities. Assure annual training programmes for the permanent staff of physicians, physicists, nurses and radiation technologists in the use of the treatment protocols to be implemented at COIL.
• Ensure continued and rapid transfer of new knowledge and advancements in the field with emphasis on safety issues.
• Ensure the integrity of medical care and application of the highest professional standards by creation of a matrix of quality control and quality assurance protocols.
Vision
Establish state-of-the-art radiation cancer treatment facilities, exploiting the unique biological and clinical features of ultra high single-dose radiotherapy, employing the most advanced standards in expertise and technology of this new mode of cancer treatment, within a caring and supportive environment.
To this end, COIL has partnered with the xxx (xxx) in xxx, a translational research institute which has pioneered SBRT and is currently developing and validating new approaches to broaden the scope of this modality in integration with molecular tumour profiling to identify the most appropriate treat
Radiobiology of Highly Hypofractionated Radiotherapy
A large body of evidence indicates that human tumors respond with high sensitivities to single-dose radiotherapy. These studies also indicate that local control is dose- dependent within a narrow dose range (20-25 Gy) regardless of tumor type (and their classical designation as “radio-resistant” versus “radiosensitive” tumors). However, the ability to treat large numbers of patients has been limited in these studies by the use of stereotactic radiosurgery, a first generation conformal radiotherapy technique that provides only limited capacity of normal tissue exclusion.
Nonetheless, the uniformity of tumor response to single dose radiotherapy regardless of tumor type supports a mechanism of response different from that regulating fractionated radiotherapy and supports the findings of experimental studies with animal models. These data also indicate that the dose range required for achieving a high rate of local tumor control (~90%) is feasible with current dose delivery systems. It should also be emphasized that single-fraction IGRT provides significant benefits in terms of convenience and potentially returning patients to systemic therapy more quickly compared with standard conventional external beam. More importantly, high dose IGRT appears to provide significantly better local tumor control compared with conventional- dose radiation, particularly for radio-resistant tumors.
Background
Cancer is a group of potentially lethal diseases, commonly characterized by uncontrolled growth and spread of abnormal malignant cells. If untreated, cancer almost invariably leads to cancer-related death. Despite the availability of curative treatments for multiple types of cancers, it has become the most common cause of death at all age groups in the western world.
The World Health Organization estimates a continuous increase in cancer incidence worldwide until 2030. The disparity between newly diagnosed cases of cancer and the annual rates of cancers deaths indicates the current anti-cancer treatments are quite effective in effectively curing an ever-growing proportion of patients. Recent improvements in 5-year survival rates reflect both progress in early diagnosis of cancer and the introduction of new and more effective anti-cancer therapies.
The introduction of computer-driven technology in radiotherapy along with the introduction of more effective and personalized systemic treatments such as targeted and anti-angiogenic therapy, have contributed significantly in the achievement of these results. While these are highly encouraging developments, they did not occur without significant cost.
These data indicate that cancer treatment continues to represent a major public health problem with significant socio-economic implications. Clearly, new effort is required to maximize the cost-effectiveness of cancer therapy to significantly reduce overall treatment costs, and improve the morbidity and long-term outcome of cancer treatments to decrease the financial impacts of lost productivity.
COIL has been designed in accordance with this vision, specifically aiming at improving the permanent control of several cancer sub-types of cancer via novel SD-IGRT technology, potentially effecting a decrease in the need for treatment of relapsing disease.
Current Treatment Modalities of Cancer
There multiple modalities routinely used in the treatment of cancer, with a certain degree of overlap in the indications for their use. Surgery, radiation therapy and systemic chemo/endocrine therapy are the main stay of cancer management.
Over the past decade, target molecules dealing with specific genomic mutations and corresponding metabolic pathways along with antiangiogenic drugs have been introduced in the management of selected disease types. While in some patients cancer can be controlled by a single modality, in particular with surgery and radiation, tumor cure frequently requires a multimodal approach given in tandem.
Recently, immunotherapy utilizing antibodies aimed at enhancing the effectiveness of the immune system against malignant cells, especially in combination with high-dose precision radiotherapy to expose tumour-specific antigens, is proving effective in increasing disease-free survival in selected groups of metastatic patients (e.g. melanoma, non-small cell lung cancer, etc). This hypothesis will be the topic of extensive research over the next years.
Surgery is primarily used for removal of visible tumor masses, although in a fraction of cases radical surgery is employed, in which removal of regional lymph nodes and/or other adjacent tissues are also performed, as clinical experience indicates that these regions may be considered at high risk of containing microscopic foci of metastatic dissemination.
Although surgery is commonly regarded as the optimal approach for the cure of localized tumors, it nonetheless often requires post-operative radiation and/or chemotherapy (adjuvant treatments) to reduce the local and distant relapse rates.
Radiotherapy may be an alternative for surgery, in particular when anesthesia and surgery represent a risk to patient’s life due to advanced age or bystander co- morbidities.
Radiotherapy is also used as primary treatment for tumors deemed unresectable by a surgical approach. As with surgery, radiation fields are frequently extended to include regional lymph nodes and/or other adjacent tissues considered at high risk of containing micro-metastatic foci. Until recently, targeting of tumors by radiation has been associated with significant levels of uncertainty, because of the inability to directly visualize tumors during treatment delivery.
Consequently, significant volumes of peritumoral healthy normal tissues were added to the treatment field as safety margins to overcome the risk missing portions by the treatment beams. Whereas at the low dose range, normal tissues are less sensitive to radiation then tumors, radiation has traditionally been delivered by the “fractionated” approach, in which daily treatments with low doses each are delivered over 3-6 weeks, gradually building up the tumor dose with concomitant reduced effect on the normal tissues.
This process is usually continued until the threshold of tissue toxicity is reached, and then stopped to avoid long-term and/or life threatening complications. However, the maximal cumulative dose feasible with fractionated radiotherapy has frequently been short of the dose required for tumor cure, resulting in local failures in nearly one third of patients treated with a curative intent.
The introduction of image guidance of radiation treatment (IGRT) over the past years, enabled by recent technological developments, has facilitated the use of visual and computer-controlled tumour targeting during treatment delivery, thus obviating the need of adding extensive safety margins of normal tissues.
A detailed account of the IRGT process and its clinical as well as logistic implications in terms shortening overall treatment times is given below. At the present time, the indications for the use of single dose or hypofractionated (up to a maximum of 5 sessions) high-precision radiotherapy have been accepted several clinical settings, in which safety and improved outcomes have been demonstrated.
COIL has been established to employ cutting-edge SDRT protocols in the many already established clinical indications and is committed to to broaden the scope of this treatment modality, whenever required in integration with other forms of highly personalized tumour-directed therapy. COIL will rapidly deploy novel modalities as they become clinically validated in new clinical indications as part of the global exclusive scientific partnership with xxx.
Chemotherapy has become increasingly popular over the last three decades due to its ability to seek and destroy microscopic foci of tumor dissemination, not detectable by CT, MRI, PET or other imaging modalities. Chemotherapy, however, increasingly fails with increasing tumour burden.
It is generally accepted that while chemotherapy confers shrinkage of visible tumors, or those large enough to be detected by imaging (≥1cm in diameter), these tumors often recur, leading to series of attempts to stop the progression of the disease using changing drug combinations and the addition of novel agents where appropriate.
While systemic therapy has become increasingly effective as a palliative modality in alleviating tumor-associated symptoms and in slowing down the progression of disease, it has also contributed significantly to the 75% rise in the cost of cancer care over the past decade.
Radiation therapy is currently going through a series of technical and conceptual revolutions that are leading to the safe delivery of radiation to unprecedented dose levels. Advances in treatment delivery accuracy currently enable the safe administration of curative treatments in a single session or in a limited number of sessions (hypofractionation).
Dose escalation to the tumor achieved through single dose or hypo-fractionated treatment regimens has brought about both an improvement in local tumor control and in patients’ survival while sparing dose to adjacent healthy tissues, thereby maximizing the therapeutic ratio and improving the patients’ quality of life.
Current research efforts are aimed at the incorporation of high-quality imaging in the radiotherapy process, both at the level of target volume delineation, with the use of state-of-the art imaging modalities for morphological and functional characterization of the tumor and at treatment delivery with the specific aim to minimize uncertainties and reduce exposure to normal tissues. This process is commonly referred to as image- guided radiation therapy (IGRT). The ultimate goal of IGRT, of course, is that radiation dose be delivered to an accurately defined target volume exactly as planned.
Indeed, 3D volumetric imaging tools for near real-time verification of target position (on-board imaging devices) are now available and appropriate correction strategies are rapidly being developed. However, image-guidance in a broader context is very much a work in progress and several important steps are in the early stages of development.
The areas which require the greatest efforts for the optimal implementation of high dose are well recognized:
(1) accurate target volume definition by state-of-the-art morphologic (MRI) and metabolic imaging (PET/CT);
(2) target motion mitigation strategies during image acquisition and treatment delivery;
(3) implementation of sound set-up correction practices. These issues have been largely resolved
The sharp dose gradient of the dose distributions associated with highly conformal IMRT treatment plans demand accurate delineation of target and surrounding critical structures at planning. Indeed, target volume delineation is likely the largest source of uncertainty in radiotherapy.
The introduction of high-speed helical scanners and the implementation of the so-called CT simulation, which minimizes systematic uncertainties has produced greater operational efficiency. The 3D data-set acquired with the patient in treatment position with appropriate immobilization devices in place, may be reconstructed through dedicated software to carry out the so-called virtual.
All of these factors have made CT simulation an integral step in modern IGRT. However, the limitations of CT imaging in the delineation of target and critical structures in many anatomical sites have become quite obvious.
Imaging for Accurate Target Delineation
Magnetic resonance imaging (MRI) provides improved soft tissue contrast, relative to CT, particularly for central nervous system structures, and within the abdominal and pelvic regions. MRI is, therefore, regarded as a fundamental imaging modality for target and critical structure delineation for intracranial, head & neck, liver and pelvic tumors. MRI by itself, through innovative algorithm which convert MR signals into CT numbers (electronic densities) are an emerging solution which obviates the use of traditional CT. Currently available image co-registration software can overcome these limitations and permit optimal use of MRI for treatment-planning.
Positron Emission Tomography (PET) has been around in the research arena for many years, and only recently has it gained widespread acceptance in the clinical practice. PET with the glucose analogue 18F-fluorodeoxiglucose (FDG) is now one of the standard methods for in vivo imaging and staging of various malignancies. The advent of PET/CT devices with co-registered functional and anatomical data has opened new exciting possibilities for target volume delineation. Extensive experience with PET/CT simulation has shown that the incorporation of metabolic information in the treatment-planning process may provide improved therapeutic ratios compared to conventional planning in many disease sites.
Increased target coverage and often reduced target volumes, in fact, may potentially result in PET/CT based planning to yield better tumor control probability through dose escalation, while still complying with dose-volume constrains (tolerance levels) for normal tissues. The optimal method to accurately determine the volume and shape of the target volume using PET information is still a matter of scientific controversy.
COIL will implement the most advanced imaging for planning purposes, incorporating metabolic information when required. Automatic artificial-intelligence-based tumour delineation and non-target tissue segmentation for treatment planning is currently being tested and requires with minimal interventions from physicians, thereby further reducing uncertainties and reducing planning times from several hours down to 15-20 minutes.
Target Motion Mitigation
Respiratory motion poses major problems in the treatment of thoracic and abdominal lesions and has traditionally been compensated by the use of a safety margin commensurate to the amplitude of motion. The large amount of healthy normal tissues exposed to high doses around the target has limited the ability to dose escalate in these regions.
The recent development of ultra-fast multi-slice CT has opened a new dimension in radiotherapy and allows time-resolved 4D CT imaging of the patient breathing cycle. The feasibility of 4D PET/CT acquisition has also recently been shown. The 4D approach obviously allows a significant tightening in the margin required, thereby reducing non- target dose and the risk of radiation-induced toxicity. Reproducibility issues at treatment delivery, however, still need to be resolved for this technique to become of routine clinical implementation.
An alternative approach that holds the promise to effectively reduce target motion due to respiration both during CT simulation and treatment delivery is respiratory gating. This system allows tracking of the tumor during treatment, with respect to the reference simulation images and turns off the beam when the target moves outside the treatment field.
Through this technology safety margins around the target may be minimized making large dose per fraction treatments feasible even in these anatomical sites. If unaccounted for, target motion may compromise the efficacy of an exquisitely crafted treatment plan.
COIL will make every possible effort to improve the therapeutic ratios of its treatments via maximal margin reduction. This will largely be achieved through the implementation of effective methods of addressing target motion both during image acquisition and treatment delivery.
Dose Delivery Assurance
Conventionally, radiotherapy treatments are delivered daily over the course of several weeks. This requires setting up the patient anew every day. Even with the highest degree of care, patient setup is not completely reproducible. Organ motion may be another source of uncertainty. In the setting of prostate cancer treatment, for instance, target position depends directly on the state of filling of the bladder and rectum which vary daily. These setup and internal motion issues are similar for almost every site in the body. Traditionally, as already mentioned, the solution to compensate for set-up uncertainties and organ motion has been to add a safety margin around the target volume.
The goal of the IGRT process is to improve the accuracy of the radiation field placement, thereby minimizing the need for additional margin and effectively reducing radiation exposure of healthy normal tissues. The key device in IGRT treatment delivery is the so- called on board imager (OBI). This apparatus is mounted on the gantry and can take images of near-diagnostic quality. Thus, a patient can be imaged directly before treatment, bony anatomy or other markers matched, and the treatment couch adjusted so as to localize the target correctly.
The information acquired through a revolution of the OBI around the patient may be used to reconstruct CT images (cone beam CT) of the patient in treatment position to be matched to the reference simulation CT images for accurate set-up correction. During the IGRT process, computer-controlled comparison between the 3D images and the reference 3D simulation images must be performed prior to treatment under the strict supervision of the treating physician.
Correction of set-up errors in near-real-time following well-defined and site-specific correction strategies can occur on a per-treatment basis obviating the need for a safety margin even in fractionated regimens (except in disease sites with intra-fraction uncertainties). This will effectively translate into reduced dose to normal tissues thus allowing dose escalation and improved local control. Indeed, the implementation of flawless set-up correction strategies is at the very heart of the philosophy of IGRT, especially when the technology is used in the context of single ultra-high dose irradiation.
The New Frontier: Single Dose IGRT
Single fraction high-dose radiotherapy (aka radiosurgery) was pioneered for brain metastasis using an invasive stereotactic frame. A large volume of clinical reports spanning decades has appeared in the medical literature to support the efficacy and safety of this approach. Indeed, at first, the use of a single fraction (stereotactic radiosurgery) or of a limited number of sessions (stereotactic radiotherapy) defied conventional wisdom in radiobiology and oncology, as hypofractionation was known to result in serious late side-effects (devascularization, fibrosis, ulceration, necrosis).
The reason that made marked hypofractionation in the brain safe and effective seems to be associated with the exquisite dosimetry (addressing almost exclusively abnormal tissues) and rigorous conduct of the treatment. This was largely dependent on the ability to fix the head precisely with well-designed stereotactic frames. As already mentioned, early experiences of hypofractionation in extracranial sites produced disappointing results due to the use of large normal tissues margins. The safe translation of single dose or marked hypofractionated regimens from the brain to the body (extracranial stereotactic radiotherapy) was essentially hindered by target motion and accuracy of target volume delineation which have been extensively described above.
Recently, the advent of IGRT has paved the way towards the implementation of such shortened treatment courses, without compromising treatment efficacy and improving its cost-effectiveness. If properly planned and delivered, IGRT enables the accurate delivery of ultrahigh doses of radiation in a single session The use of this approach, however, requires specific expertise in the design and delivery of treatment at extreme levels of precision, as there is no tolerance for error with these treatments.
Radiobiology of Highly Hypofractionated Radiotherapy
While the radiobiology of conventionally fractionated radiotherapy has now largely been unraveled and the bulk of the radiation damage is attributable to double DNA strand breaks, little is still known about the mechanism of action of large fraction size irradiation.
The mechanism of tumor response after single-dose radiotherapy differs from that of fractionated radiotherapy. Recent studies indicate that single high-dose radiation exposure engages a microvascular component in tumor response. This response is engaged in the clinical realm at dose levels in the range of 20-25Gy. This mechanism, therefore, would not be activated by fractionated radiation schemes.
SUMMARY OF TERMINOLOGY
Brain Metastasis
Cancer that has spread from the original (primary) tumor to the brain.
Chemotherapy
Treatment that uses drugs to stop the growth of cancer cells, either by killing the cells or by stopping them from dividing. Chemotherapy may be given by mouth, injection, or infusion, or on the skin, depending on the type and stage of the cancer being treated. It may be given alone or with other treatments, such as surgery, radiation therapy, or biologic therapy.
CT Scan
A procedure that uses a computer linked to an x-ray machine to make a series of detailed pictures of areas inside the body. The pictures are taken from different angles and are used to create 3-dimensional (3-D) views of tissues and organs. A dye may be injected into a vein or swallowed to help the tissues and organs show up more clearly. A CT scan may be used to help diagnose disease, plan treatment, or find out how well treatment is working. Also called CAT scan, computed tomography scan, computerized axial tomography scan, and computerized tomography.
Endocrine Therapy
Treatment that adds, blocks, or removes hormones. For certain conditions (such as diabetes or menopause), hormones are given to adjust low hormone levels. To slow or stop the growth of certain cancers (such as prostate and breast cancer), synthetic hormones or other drugs may be given to block the body’s natural hormones. Sometimes surgery is needed to remove the gland that makes a certain hormone. Also called hormonal therapy, hormone therapy, and hormone treatment.
Hypofractionated Radiation Therapy
Radiation treatment in which the total dose of radiation is divided into large doses and treatments are given once a day or less often. Hypofractionated radiation therapy is given over a shorter period of time (fewer days or weeks) than standard radiation therapy.
Image Guided Radiotherapy
A procedure that uses a computer to create a picture of a tumor to help guide the radiation beam during radiation therapy. The pictures are made using CT, ultrasound, X-ray, or other imaging techniques. IGRT makes radiation therapy more accurate and causes less damage to healthy tissue. Also called image-guided radiation therapy.
Immunotherapy
A type of biological therapy that uses substances to stimulate or suppress the immune system to help the body fight cancer, infection, and other diseases. Some types of immunotherapy only target certain cells of the immune system. Others affect the immune system in a general way. Types of immunotherapy include cytokines, vaccines, bacillus Calmette-Guerin (BCG), and some monoclonal antibodies.
Metastasis
The spread of cancer cells from the place where they first formed to another part of the body. In metastasis, cancer cells break away from the original (primary) tumor, travel through the blood or lymph system, and form a new tumor in other organs or tissues of the body. The new, metastatic tumor is the same type of cancer as the primary tumor. For example, if breast cancer spreads to the lung, the cancer cells in the lung are breast cancer cells, not lung cancer cells. The plural form of metastasis is metastases.
MRI Scan
A procedure in which radio waves and a powerful magnet linked to a computer are used to create detailed pictures of areas inside the body. These pictures can show the difference between normal and diseased tissue. MRI makes better images of organs and soft tissue than other scanning techniques, such as computed tomography (CT) or x-ray. MRI is especially useful for imaging the brain, the spine, the soft tissue of joints, and the inside of bones. Also called Magnetic Resonance Imaging, NMRI, and nuclear magnetic resonance imaging
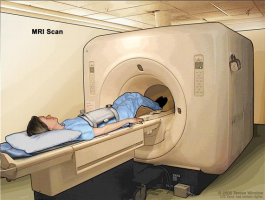
a table that slides into the MRI machine, which takes pictures of the
inside of the body. The pad on the patient’s abdomen helps make the
pictures clearer.
PET Scan
A procedure in which a small amount of radioactive glucose (sugar) is injected into a vein, and a scanner is used to make detailed, computerized pictures of areas inside the body where the glucose is taken up. Because cancer cells often take up more glucose than normal cells, the pictures can be used to find cancer cells in the body. Also called Positron Emission Tomography scan.
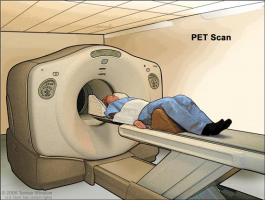
slides through the PET machine. The head rest and white strap help the
patient lie still. A small amount of radioactive glucose (sugar) is injected
into the patient’s vein, and a scanner makes a picture of where the
glucose is being used in the body. Cancer cells show up brighter in the
picture because they take up more glucose than normal cells do.
PET-CT Scan
A procedure that combines the pictures from a positron emission tomography (PET) scan and a computed tomography (CT) scan. The PET and CT scans are done at the same time with the same machine. The combined scans give more detailed pictures of areas inside the body than either scan gives by itself. A PET-CT scan may be used to help diagnose disease, such as cancer, plan treatment, or find out how well treatment is working. Also called positron emission tomography-computed tomography scan.
Primary Tumour
A term used to describe the original, or first, tumor in the body. Cancer cells from a primary tumor may spread to other parts of the body and form new, or secondary, tumors. This is called metastasis. These secondary tumors are the same type of cancer as the primary tumor. Also called primary cancer.
Radiosensitisation
The use of a drug that makes tumor cells more sensitive to radiation therapy.
Radiosensitive
Being unresponsive to radiotherapy
Stereotactic Body Radiation Therapy
A type of external radiation therapy that uses special equipment to position a patient and precisely deliver radiation to tumors in the body (except the brain). The total dose of radiation is divided into smaller doses given over several days. This type of radiation therapy helps spare normal tissue.
Leave a Reply